Crank Length & Bike Fit
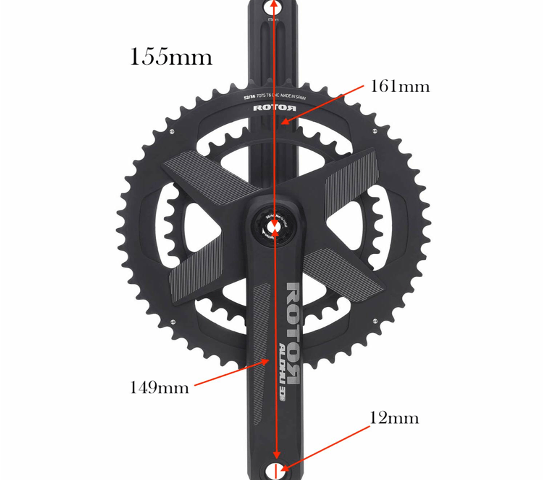
Background
My mountain bike racing ‘career’ started in New England during the late 80’s riding 175mm crank arms. Nearly all mountain bikes were outfitted with that size. The tendency to change was typically longer (180mm) instead of shorter (170/167.5/165). We all knew a longer arm was “better” because it produced more leverage/torque on the drivetrain (which is true). My brother (6’5”) used 180’s. I wanted to be like him, so I tried 180’s too, but could not find a position that was tolerable for my knees.
I moved to Pittsburgh for grad school in the early-90’s where I rode messenger in addition to racing MTB and criterium – all on 175mm cranks. I was riding 20k miles a year, placing top-10 at most elite mountain bike and criterium competitions without symptoms.
Unfortunately, the progression of Lyme disease that I had contracted a few years earlier in Connecticut started taking a toll on my knees. I was unwilling, at the time, to try shorter cranks despite this being my recommendation for my bike fit-clients with similar symptoms. By 1994 I had to stop riding. It was the hardest thing I have ever done in my life.
Nearly a year later while collecting parts for my sister’s retro-bike on the 2nd floor of Kraynick’s bike shop, I literally stumbled into a set of beautiful, vintage 170mm 42/53 Campy cranks. He charged me $30, so I figured why not? Installed a campy taper bottom bracket, raised the saddle 5mm to offset the change from 175-170 rode 60-miles without a hint of knee pain.
Within 3-months my power was back, but being a type “A” personality made that time grueling. The combination of being out of shape, Lyme symptoms, and a change in crank length required that I start from scratch with my training.
It should be clear by now that I was a bike freak. So much so, that I dropped out of my doctorate program, put my master’s degree away and moved to Minneapolis where I started Erik’s “True Fit” in 1999. My passion for research never waned – I just shifted focus from epidemiology to biomechanics and neurology. I’ve managed to allocate at least 10-hours per week to research since I l left the doctoral program. Starting from scratch, being essentially self-taught has proven more effective than being in school, because my research was specific to problem solving instead of getting a degree. I enjoy testing research findings against my interventions/outcomes, and identifying the flaws in methodology or application when there is disparity between research and practical application.
I went solo with Bicycle Fit Guru in 2007, investing thousands in 3D equipment, sEMG, fit bikes, sMo2, high speed video, etc., which I used to further research. My subject pool consisted of about 19-elite and novice athletes that I sponsored until 2012. We met monthly for integrated 3D/sEMG assessment, position refinement, pedaling efficiency training, yoga, meditation, and/or whatever an individual needed to go faster.
Jim Martin et. al. published a very well written article around that time, suggesting crank lengths between 145-190 had no impact on max power output for cyclists of varied height and morphology. His was not the first. I mostly ignored these findings because crank length discussions suck, and I wanted to preserve my reputation. I’d put short cranks on a bike to reduce symptoms, but only as a last resort to avoid hampering performance.
John Cobb started pushing short cranks for triathletes, and I followed suit. His logic was sound, though in my experience there was an accommodation period extending from 30-90-days after installation. I tried using sEMG when adjusting to maintain similar lower body muscle recruitment patterns to no avail. Position changes did not require modifying cockpit stack relative to saddle height in most cases, though saddle X/Y varied between individuals. We did not observe changes in bike split time, but virtually ALL athletes ran better off the bike. I suspect reduced inspiratory muscle fatigue and cardiac output contribute to the improved run, but sMo2 levels were higher at the deltoids (and other trunk muscles) which suggests a better utilization of oxygen (legs), but that is just a guess.
Greg LeMond reached out in 2012 to discuss some business ideas. During our meeting, he asked me what I thought about crank length. I described what I’d seen with triathletes, but suggested that benefit or lack thereof was entirely psychological aside from addressing symptoms/counterproductive pedaling. He informed me that he was using 145mm cranks and was able to demonstrate power equal to his racing days with only 2-weeks high interval training (might be a key to reducing accommodation latency?). Next, he asked the question, “would you rather do a shallow or deep squat”, which really puts the “longer lever” argument to rest. In hindsight, I probably should have asked what he thought before saying anything.
Ego Killer
“There is a principle which is a bar against all information, which is proof against all
argument and which cannot fail to keep a man in everlasting ignorance.
This principle is contempt prior to investigation examination.”
Herbert Spenser.
Action
The next day I bought a pair of 155mm Rotor In-Power cranks, installed them, raised the saddle and moved it back a few mm’s to maintain joint moments relative to the bottom bracket and went for a ride. I was riding faster, but producing less power regardless of how many times I recalibrated the unit. My CIDP (large fiber demyelination) – the gift of Lyme that keeps on giving – appeared to benefit from the reduced ROM, probably because it required less motor control.
Thought to myself this was great for flats, but standing, climbing, would suffer due to the shift from joint moments to mass for crank rotation. Nope. Felt slower, but speed remained unchanged. My best guess to explain this phenomenon is that the step – like climbing stairs — is smaller with the shorter cranks – 13.7” (175mm) vs. 12.2” (155mm).
I still ride 155’s on my road bike. My power returned after a few months and I enjoy the higher cadence. I’ve tested 155’s on my mountain bike and was unwilling to spend the time re-learning how to handle technical sections with a shorter stance. Appleman makes short fat bike cranks, and I plan on testing 145mm soon. Hoping the reduced ROM will minimize counter steering and improve balance in soft snow.
Mission
I’ve spent the past 10-years of my career refining my understanding of the benefits/costs of changing crank length for my clients. Less than .02% (2 of more than 1,000) rejected short crank conversions. One was told by a PT bike fitter that longer cranks would reduce lateral knee symptoms; the other was unable to produce the same power with 165 as 170 within 2-months
Research
James C Martin, PhD, at the University of Utah Neuromuscular Function Lab, is one of the most prominent names in crank length studies.
I’m citing his work specific to the impact of crank length on maximum power output, metabolic cost, and fatigue.
Martin’s findings suggest differences in max power between 145mm and 190mm crank lengths are minimal, with 145 producing higher output than 170mm and 190mm. 120mm and 220mm cranks appeared to compromise maximum power significantly.
He also identified a correlation between pedal velocity and metabolic cost, which most of us have experienced – pedal faster and breath harder, etc. In fact, increased foot velocity – not cadence – is correlated to metabolic cost. Foot velocity is the speed that the foot travels in space, which is slower with shorter cranks even at a slightly higher cadence because the distance traveled is reduced.
And muscle fatigue did not appear impacted by crank length when pedaling at a fixed cadence, indicating fatigue is most likely related to contraction cycles — not crank length.
Studies
(not exhaustive)
These are some of the more popular research papers on crank length. There are numerous flaws throughout, including but not limited to sample size and representation, duration, using the Wingate test, not changing saddle or handlebar X/Y relative to changes in crank length, etc. All research has flaws. What matters is that the findings remained consistent, even as these flaws were recognized and addressed in later papers.
Barret P, Korff T, Elmer S, Martin J (2011) Effect of crank length on joint-specific power during maximal cycling. Med Sci Sports Exerc. 43(9):1689-97.
Lengths tested: 150mm-190mm.
Findings: No effect on joint specific power at hip, knee and ankle between 165-175mm; small but significant decrease in hip power using 150mm compared to 190mm.
Barratt PR, Martin JC, Elmer SJ, Korff T. Effects of pedal speed and crank length on pedaling mechanics during submaximal cycling. Med Sci Sports Exerc. 2016 Apr;48(4):705-13.
Lengths tested: 150mm-190mm
Findings: Increased knee and hip angular excursion with longer crank lengths. Crank length did not impact moments or power at the ankle, knee and hip.
Burrus BM, Armendariz J, Moscicki BM (2021) Cycling with short crank lengths improved economy in novices. Int J Exerc Sci. (1):1123-1137.
Lengths tested: 175, 145
Findings: Improved power, economy and decreased pedal speed (foot velocity not cadence) in the 145mm condition.
Conrad DP (1983) Bicycle crank arm length and oxygen consumption
in trained cyclists. Med Sci Sports Exerc 15(2):111–112.
Lengths tested: 165mm-180mm, in 2.5mm increments.
Findings: No correlation between crank arm length and limb segments (length) relative to VO2 values, and no significant differences in VO2 between crank arm lengths.
Hull ML, Gonzalez H (1988) Bivariate optimization of pedalling rate
and crank arm length in cycling. J Biomech 21(10):839–849
Lengths tested: 140mm-200mm
Findings: 170mm @ 100rpm corresponds closely to the cost function minimum. Optimal pedaling rate crank arm length is 110rpm using 145mm cranks. with longer cranks increasing hip moments and kinematics relative to knee and ankle joints.
Martin JC, Spirduso WW (2001) Determinants of maximal cycling
power: crank length, pedalling rate and pedal speed. Eur J Appl
Physiol 84:413–418
Lengths tested: 120-220.
Findings: Max power produced with 145mm cranks, followed by 170mm. Optimal length proposed = tibia x .41.
Inbar O, Dotan R, Trousil T, Dvir Z (1983) The effect of bicycle
crank length variation upon power performance. Ergonomics
26(12):1139–1146
Lengths tested: 125-225(125, 150, 175, 200, 225)
Findings: Highest mean power and max power @ 155mm cranks (16.4-16.6 Calculated from the best fit parabolic curve).
Macdermid, PW, Edwards, A (2010) Influence of crank length on cycle ergometry performance
of well-trained female cross-country mountain bike Eur J Appl Physiol (2010) 108:177–182
DOI 10.1007/s00421-009-1197-0
Lengths tested: 170-175
Findings: Decreased time to peak power using 170mm vs 172.5mm & 175mm cranks – no changes in other power/performance
Morris DM, Londeree BR (1997) The effects of bicycle arm length on
oxygen consumption. Can J Appl Physiol 22(5):429–438.
Lengths tested: 165-175.
Findings: Decreased oxygen consumption with 165mm cranks vs. 170mm and 175mm. No, predictive value in leg length to determine optimal crank length.
Too D, Landwer GE (2000) The effect of pedal crank arm length on
joint angle and power production in upright cycle ergometry.
J Sports Sci 18:153–161
Lengths tested: 110-265 (110, 145, 180, 235, 265)
Findings: Changes in crank length impacted knee ROM (max/min 30.5-deg.) more than hip ROM (max/min 14.3-deg.) greater than hip angle. Also, that the ankle position shifted from dorsifexed position to plantarflexed as crank length increased. 180mm cranks produced the highest power. This study is an outlier re: optimal crank length – probably due to the upright cycling position.
Biomechanics Perspective
It is true that a crank arm produces rotational torque on a bicycle drivetrain, but the motor moving that arm is our body.
There are three primary joints producing power when cycling – the hip, knee and ankle. Muscles crossing these joints create forces (torque) on the bones (levers) to produce extension and flexion (rotation).
This radically oversimplified diagram demonstrates what happens when a cyclist pushes down on the bicycle pedal.
- Muscles have origins and insertions. The amount of torque a muscle can produce (joint rotation) is determined by the distance between the center joint, perpendicular to the muscle line of pull (moment arm). As you can see from the image, the glutes/hamstrings can produce more torque on the hips than the quadriceps on the knee.
- Each muscle has a line of pull. It is rarely direct, and much is dictated by pinnation. The sagittal line of pull for the quadriceps appears simple, but these muscles meet at the patella which is attached to the tibia by the patella tendon.
- Effective force (net joint moments) causes the rotation that occurs at a joint. This is what makes the leg extend so you can push into the pedals. Peak muscle activation is not equal to peak muscle torque. This is determined by the type of lever (class) moment arm and joint angle.
- Ineffective force is a byproduct effective force or torque. This is greatest between the patella and femur when cycling. The quadriceps create tension on the patellar tendon, compression between the patella and femur and shearing when the patella pulled out of alignment.
This graphic demonstrates the complexity of muscle recruitment at the knee joint.
Crank Issues
Most of us assume that pedals travel in a perfect circle. They do not. The reason for this is that the pedal axle is between the bottom bracket and pedal platform at the top of the pedal stroke, which increases the distance by about 12mm. This has a negative impact on pedaling inertia (external force) and compressive forces at the hip (internal force). I also believe this is why eliminating the compressive force at the hip tends to reduce crank and pedal based power readings more than rear wheel and/or direct drive power systems.
Crank Length and Joint Angles
The only changes made to this position included raising the saddle equal to the difference in crank length and shortening the stem to account for a more setback seat position. This is not an accurate representation of the position changes required with shorter cranks, but it is a good place to start and demonstrates my point. Reducing knee flexion when cycling has a positive impact patellofemoral compression because all quadriceps muscles attach to the patella (knee cap). This is more effective for patellofemoral (under the knee) issues than tibiofemoral (between the femur and tibia) issues though it appears to benefit both.
Regarding Crank Length Formulas
Thre is no 100% accurate formula for determining the correct crank length for an individual, due to the numerous confounding variables inherent to individuals, positioning, and technique.
Here are a few of the big ones:
Femur to Tibia Ratio
Disscusions regarding optimal crank length selection tend to focus on femur length. Femur length matters far less than tibia length as the basis of optimal crank selection. The tibia is more vertical than the femur, and therefore has a greater impact on hip range of motion and patellar compression at top dead center when pedaling.
The rider on the left has a greater femur to tibia ratio than the right. Overall leg-length is identical, but the hip angle varies by ten-degrees.
Ankling Pattern
Individuals exhibit unique ankling patterns. The ankle angle at TDC has a profound impact on joint angles, which impacts muscle recruitment and joint forces.
The rider on the right has increased the virtual crank length by more than 3cm at TDC.
Lumbar Spine
The lumbar spine has a large impact on hip range of motion when cycling. A flat or lordotic lumbar spine reduces functional/cycling hip range of motion because it rotates the pelvis anteriorly, driving the inferior aspect of the anterior superior iliac spine (ASIS) towards the proximal femur.
There are greater net joint force (total force) at the hips and knees for the rider on the left, and greater net joint moments (rotational force) at the hips and knees for the rider on the right. This is compounded for the rider on the left if they are exceeding functional hip and/or knee range of motion.
Off bike hip flexibility testing is a poor predictor of hip range of motion on a bicycle because the spinal extensors are not active A lordotic spine (think good posture) reduces hip range of motion more than a kyphotic or flexible lumbar spine (look at professional cyclists).
As good as lumbar lordosis is for life, it can be a liability on the bike.
A reminder to the PT’s reading this blog: cycling is not a natural movement pattern – it is accommodative, always. Applying human postural biomechanics to cycling postural biomechanics is illogical.
Bike Fitting
I do not have a short crank fetish. In fact, the only time that I suggest a reduction in crank length is when the current length is producing a negative impact on pelvic stability (or symptomatic). My approach to fitting targets pelvic stability as the foundation for all adjustments, and necessary to establish a positive outcome and successful training.
Pelvic stability is also the baseline for productive pedaling. Hip rocking as pelvic rotation is not a problem when it demonstrates force production. It is natural and good for the pelvis to rotate when pedaling – especially common with increased power production. Pelvic stability increases differentiation between upper and lower body mechanics, which improves oxygen delivery to the glute and leg muscles.
Pelvic instability correlates to counterproductive pedaling, where the body is pulled or pushed during crank rotation. Sticking with long cranks here, If the pelvis is displaced from the saddle at TDC – the entire body is in a compensatory pattern in response to that movement. If the one hip is displaced at TDC, the opposing arm is forced to brace against that force. As the hip returns to “neutral” the weight of the body compresses the bones against the saddle, typically accompanied by ipsilateral lumbar extensor and arm recruitment. These compensatory patterns increase upper body recruitment patterns, which diverts oxygen from the glute and leg muscles.
The baseline for productive pedaling assumes the body is not being pulled or pushed by the pedal. It is common to identify hip “rocking” as a saddle being too high, though the hips will also rock when the cranks are too long.
Symptoms
I am going to list a few of the more common symptoms associated with improper crank length. This is not exhaustive. Furthermore, I cannot guarantee a reduction in symptoms moving to shorter cranks because I have no way of ensuring your are fit properly and/or that your condition is entirely cycling related.
- Knee pain. Typically patellofemoral or at the patella tendon. Lateral or medial due to muscle pull or when knee tracking is windswept to accommodate restricted hip range of motion at TDC
- Back pain. Bilateral (unilateral is typically imbalance). Exceeding functional hip ROM can produce a posterior pelvic rotation that strains the lumbar extensors. Bouncing as a byproduct of this also tends to increase lumbar extensor recruitment patterns/fatigue.
- Saddle discomfort/saddle sores. What goes up must come down. Each time your butt hits the saddle it pulls hair follicles, opens pores and irritates the periosteum.
- Upper body pain. Variable. We are not symmetrical organisms. Bracing against pelvic displacement can manifest anywhere in the upper body.
- Glute recruitment. Yup. In my experience using sEMG, this displacement/bouncing appears to inhibit glute max recruitment pedaling.
Testing
Have your bike position established by a competent fitter so you can isolate the variable “crank length” when testing. I suggest having that person conduct these tests to ensure objectivity.
- Global assessment. Sagittal view of the hip using video camera and software with tracking capabilities. Place a tracker on the greater trochanter and observe the amount of displacement when pedaling. If the hip continues to rise after max hip flexion they are exceeding functional ROM. This assumes proper saddle height is established to ensure the contralateral leg is not lifting the observed hip at BDC.
- Hip. The simplest test for functional hip ROM issues is to slide a finger into the crease of the hip, between the femur and inferior aspect of the ASIS. Shorter cranks are indicated if your thumb is crushed between these two-bones at TDC. Moving the saddle forward and raising the handlebars can impact this variable, but I am assuming those contact points are already optimal.
- Knee & Ankle. Reverse pedaling. Pedaling backwards is a popular trick for testing crank length, where angular deceleration approaching TDC indicates exceeding hip ROM. I prefer use this test for knee and ankle ROM issues because functional hip ROM is impacted by glute recruitment which is absent when reverse pedaling.
- Hands on. Place your fingers on the posterior pelvis where it meets the sacrum — the superior aspect of the sacroiliac joint. Check for excessive distraction or compression. You will feel the space increase with distraction and it tighten while the hip is still flexing with compression. This is better suited for professionals who can differentiate between nutation/counternutation and compression/distraction.
Questions
If biomechanics are improved with a shorter crank arm why does power remain nearly identical between different conditions?
Do crank and pedal based power systems register power when a cyclist exceeds joint (hip, knee, ankle) ROM as a function of increased tangential pedal loads on the downstroke from bouncing?
Does the reduction in knee moments associated with shorter cranks increase hamstring moments, more closely approximating Lombard’s paradox?
What is the role of psychology in crank length effectiveness?
How is it that some cyclists experience no accommodation period with changes in crank length and others require up to three-months?
What mechanism explains nearly identical findings between two-studies, where one adjusted saddle and bar height relative to changes in crank length and the other did not?
Is the reduced cardiac output associated with shorter cranks at similar cadence responsible for the apparent running gains in triathlon?
How does the reduction in stance – distance between front and rear foot – with short cranks impact stability when standing, mountain biking?